UC San Diego Researchers Build First 500 GHz Photon Switch
Published Date
By:
- Doug Ramsey
Share This:
Article Content
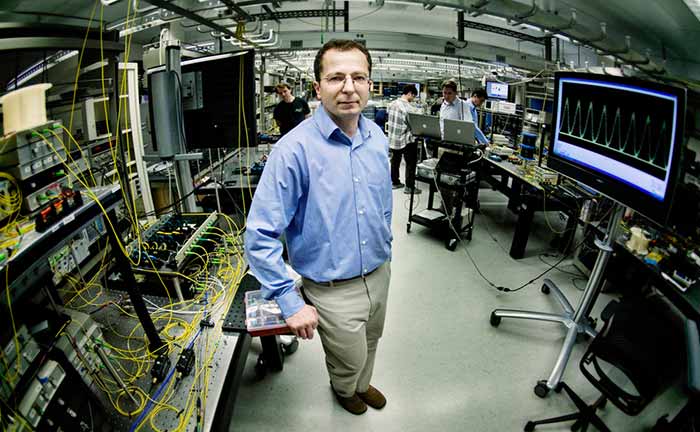
Electrical and Computer Engineering professor Stojan Radic in the Photonic Systems Laboratory of the Qualcomm Institute
The work took nearly four years to complete and it opens a fundamentally new direction in photonics – with far-reaching potential consequences for the control of photons in optical fiber channels.
Researchers at the University of California, San Diego have built the first 500 Gigahertz (GHz) photon switch. “Our switch is more than an order of magnitude faster than any previously published result to date,” said UC San Diego electrical and computer engineering professor Stojan Radic. “That exceeds the speed of the fastest lightwave information channels in use today.”
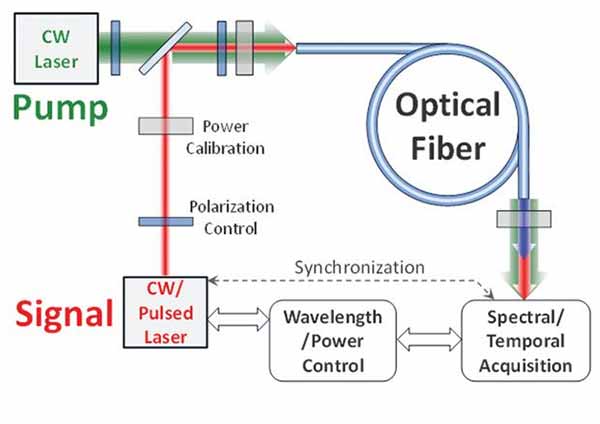
Schematic for static and fast switching characterization: A continuous-wave (CW) laser pump combined with classically attenuated weak CW or pulsed signal, launched into the nonlinear fiber.
According to an article in the journal Science*, switching photons at such high speeds was made possible by advances in the control of a strong optical beam using only a few photons, and by the scientists’ ability to engineer the optical fiber itself with accuracy down to the molecular level.
In the research paper, Radic and his colleagues in the UC San Diego Jacobs School of Engineering argue that ultrafast optical control is critical to applications that must manipulate light beyond the conventional electronic limits. In addition to very fast beam control and fast switching, the latest work opens the way to a new class of sensitive receivers (also capable of operating at very high rates), faster photon sensors, and optical processing devices.
To build the new switch, the UC San Diego team developed a new measurement technique capable of resolving sub-nanometer fluctuations in the fiber core. This was critical because local fiber dispersion varies substantially, even with small core fluctuations, and until recently, control of such small variations was not considered feasible, particularly over long device lengths.
In the experiment, a three-photon input was used to manipulate a Watt-scale beam at a speed exceeding 500 Gigahertz.
In their research, the engineers in the Photonic Systems Laboratory of UC San Diego’s Qualcomm Institute demonstrated that fast control becomes possible in fiber made of silica glass. “Silica fiber represents a nearly ideal physical platform because of very low optical loss, exceptional transparency and kilometer-scale interaction lengths,” noted Radic. “We showed that a silica fiber core can be controlled with sub-nanometer precision and be used for fast, few-photon control.” Until recently, control of small variations was not considered feasible – particularly over long scales. But once they were able to profile the fluctuation of the actual fiber, it became clear that the silica fiber core could be controlled with sub-nanometer precision – and be used for fast, few-photon control.
Controlling a strong optical beam is not easy. Departing from the conventional approaches that rely on highly resonant physical processes or optical cavities to control an optical beam directly, the UC San Diego team used specially designed, highly nonlinear fibers and they generated all the pulses necessary for the experiments. To design the new switch, they had to derive new theory to describe interaction between photons in fiber core controlled at molecular scale. To build the device, the team also developed a new measurement technique capable of resolving sub-nanometer fiber core fluctuations. “We were able to use the technique to synthesize the first photon gate actuated by only three photons at 500GHz,” said Radic, adding that two key contributors to synthesizing the photon gate include “the ability to predict the optimal microscopic variation, and our ability to measure such variations in physical fiber.”
Fibers may look identical to conventional measurement instruments, and even possess the same standard core variation, yet they may offer dramatically different switching performance. This is primarily due to extreme sensitivity to core fluctuations. (To be used for switching and processing, photons must interact with each other, whereas photons used to communicate information through long-distance fiber travel in a vacuum and do not interact.)
Sensitivity to fluctuations is particularly noteworthy in the context of the fiber’s core structure, which is traditionally made of glass. Its basic building block, the silicon-oxide (Si-O) molecular ring, has a 0.6-nanometer diameter, and it defines the ultimate precision with which a physical fiber core can be realized. Until recently, control of small variations was not considered feasible – particularly over long scales. But once the researchers were able to profile the fluctuation of the actual fiber, it became clear that a silica fiber core could be controlled with sub-nanometer precision, and be used for fast, few-photon control.
What made it not just feasible, but practical, was progress in measuring fiber with sub-nanometer precision. They came up with a way to measure lengths of fiber without doing damage to the fiber. “We measured kilometers of fiber samples and recorded the core variations,” said Nikola Alic, a research scientist in the Photonic Systems Laboratory. Added Radic: “The technique is so sensitive, that if a fly landed on a fiber many miles away, it would distort the core ever so slightly – and we could detect and measure it.”
After measuring the fiber, the UC San Diego researchers were able to generate what they call a “nanoscale signature library.” From there, they identified a specific core fluctuation profile that would correspond to the maximum depletion of the photon pump. Once the calculation yielded a unique core variation profile for a length of fiber, the scientists combined two distinct fiber sections from the core fluctuation library with the same variation profile.
Then came the hard part: figuring out the efficiency and speed of few-photon control. They were able to estimate the minimum number of photons in the control pulse permitted by the specific fiber. The resulting 2.5 picosecond-long pulse (one second equals one trillion picoseconds) with a peak power of 178 nW, contains less than three photons – indicating the feasibility of few-photon switching at a 500GHz rate.
“We addressed the feasibility of few-photon switching in locally controlled fiber,” said Radic, “and it is not very difficult to predict the broader implications of this approach. Specifically, the technology could be implemented for photon sensors that operate in fields that were previously not deemed possible based on the current technology roadmap.” An example is a receiver that could detect a handful of photons but very slowly – with the time delay between such pulses on the order of nanoseconds, not picoseconds (one nanosecond equals 1,000 picoseconds).
Another example: long-scale, locally-controlled four-photon mixing may trigger a multi-frequency photon avalanche, meaning that a few-photon signal could induce massive pump photon annihilation.
To take full advantage of photon switching, says Radic, a new class of fibers is needed – fibers in which the fluctuation of stochastic (randomly determined) dispersions could be minimized. These must be engineered fibers, and the UC San Diego team has already built a first prototype of a fiber engineered for this purpose.
*R. Nissim, A. Pejkic, E. Myslivets, B.P. Kuo, N. Alic, S. Radic, “Ultrafast optical control by few photons in engineered fiber,” Science, pp. 417-419, Vol. 345, Issue 6195, 25 July 2014. DOI:10.1126/science:1253125
Share This:
Stay in the Know
Keep up with all the latest from UC San Diego. Subscribe to the newsletter today.