By:
- Heather Buschman
Published Date
By:
- Heather Buschman
Share This:
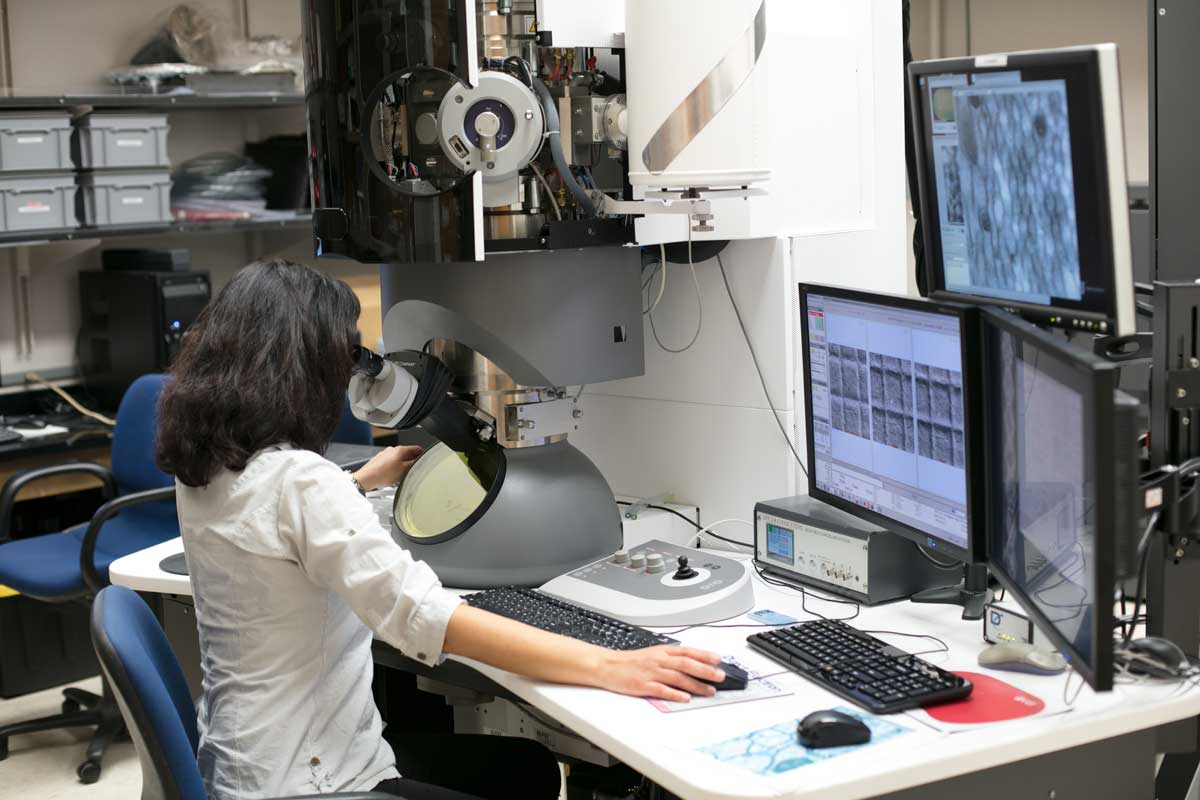
The National Center for Microscopy and Imaging Research (NCMIR) at UC San Diego School of Medicine is an international resource for microscopy expertise, infrastructure, technology development and analysis.
Seeing is Understanding
The way we see cells has changed dramatically in the last 50 years
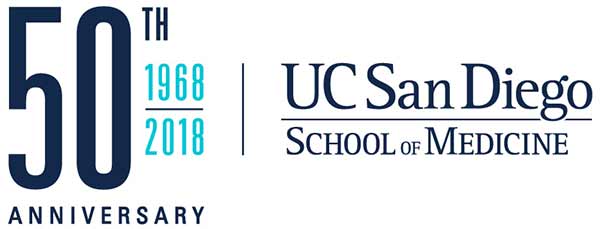
In the 1980s and ’90s, Roger Tsien at UC San Diego School of Medicine and three colleagues on the other side of the country made the invisible visible.
Osamu Shimomura had long studied bioluminescent jellyfish at the Marine Biological Laboratory in Woods Hole, Mass., and first identified the green fluorescent protein (GFP) that helps it glow. Douglas Prasher, also working at Woods Hole at the time, then isolated the gene that encodes GFP. Prasher sent the gene to Martin Chalfie at Columbia University, who used it to illuminate human and other cells.
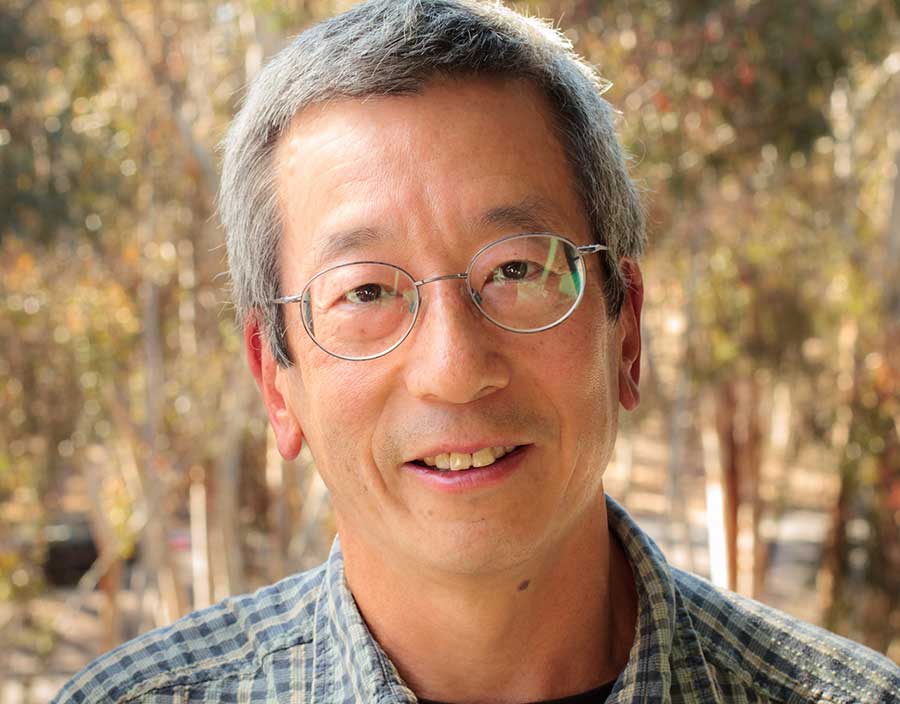
Roger Tsien shared the 2008 Nobel Prize in Chemistry for his work developing green fluorescent protein (GFP) and its colorful cousins into ubiquitous imaging tools.
Prasher also sent the GFP gene to Tsien, who took their work a step further. Combining his skills in chemistry and biology with new genetic engineering technologies, Tsien found ways to tweak GFP’s makeup, making it glow more brightly and consistently. Then, he created a full color palette of fluorescent proteins that scientists could use to track different cellular processes at the same time. He then used different color combinations that can report changes in a specific molecule’s concentration and location within cells.
GFP and its rainbow of cousins with names like mCherry and mOrange quickly became indispensable tools in life sciences labs around the world, allowing researchers to look into cells or whole animals, to watch molecules interact in real-time and ask questions once thought impossible.
“Our work is often described as building and training molecular spies,” Tsien once said, “molecules that will enter a cell or organism and report back to us what the conditions are, what’s going on with the biochemistry while the cell is still alive.”
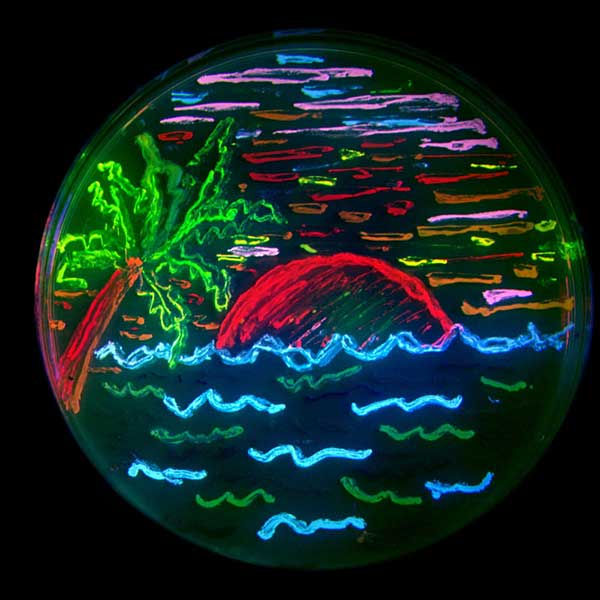
Roger Tsien developed a colorful array of fluorescent probes, including those used here to illuminate bacteria growing in a petri dish.
In 2008, Tsien, Shimomura and Chalfie were awarded the Nobel Prize in Chemistry. The Nobel committee highlighted a few of GFP’s many applications, such as tracking the development of Alzheimer’s disease in the brain or the growth of pathogenic bacteria.
As professor of pharmacology, chemistry and biochemistry at UC San Diego School of Medicine, Tsien continued his research, mentored students and postdoctoral trainees and taught classes for 27 years.
When he died in 2016, social media literally illuminated in his memory, with scientists around the world posting images of cells glowing in his honor.
Tsien’s legacy lives on. His work forms the basis for untold numbers of laboratory and clinical technologies and discoveries. Two described here are ongoing at UC San Diego School of Medicine — an effort to make surgeries safer and more precise and laboratory studies of how memories are stored in the brain.
Enlightened surgery
Surgeons often rely on feel, look and experience to tell if they have removed all of a tumor while sparing healthy tissue and nerves. Unfortunately, this inexact approach means tumor tissue is sometimes missed, increasing the chances the malignancy will reoccur and the patient will require further treatment. In some cases, incidental nerve damage during surgery can cause weakness, pain or even paralysis.
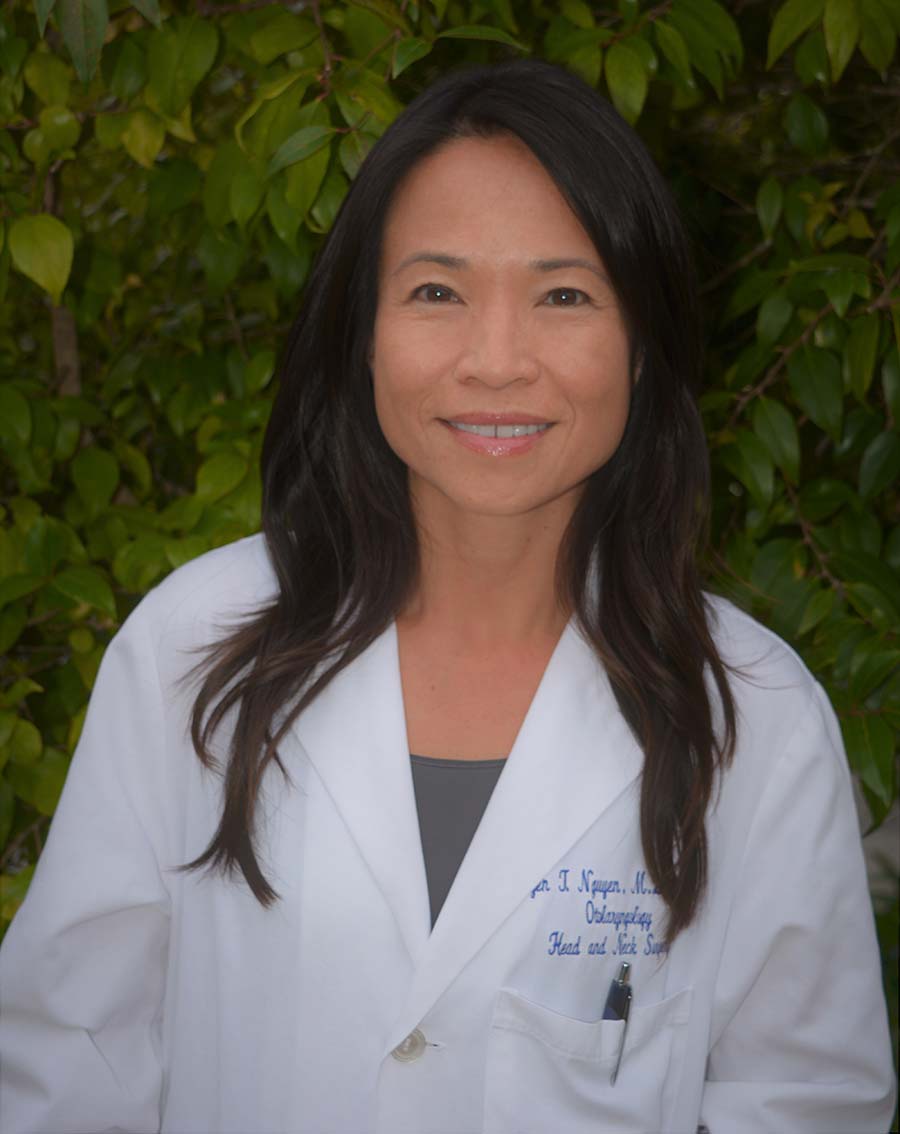
Dr. Quyen Nguyen, professor and director of the Facial Nerve Clinic at UC San Diego Health
To see if they might be able to make surgery safer and more effective, Tsien teamed up with Dr. Quyen Nguyen, head and neck surgeon at Moores Cancer Center at UC San Diego Health. Over 13 years of collaboration, they developed injectable fluorescent molecules that specifically light up tumors and hard-to-see peripheral nerves.
Here’s how the tumor-labeling molecule works. First, the molecule is U-shaped and comprises three parts. The first is a positively charged polycation, which sticks to every tissue. The second is a negatively charged polyanion, which is non-sticky and so acts like the back of a sticker. The third is a cleavable link that can only be cut by the right enzyme in the body, which acts like very specific molecular scissors.
When the three-part dye hits normal body tissue, nothing happens. But cancer cells have molecular scissors that snip the link, removing the backing from the sticker and causing the tumor to radiate with fluorescent dye.
“The cool thing about this fluorescence is not just that it’s bright, but that it can go through tissue, so you can see a tumor even if it’s buried deep in other tissue,” Nguyen said. “Cancerous tissue just glows, right there in the operating field.”
Tsien, Nguyen and colleagues also screened thousands of small peptides (amino acid chains, like those that make up proteins) for those that preferentially bind peripheral nerve tissue in mice. They labeled the most promising candidate with a fluorescent tag and found that it creates a distinct contrast — up to tenfold — from adjacent non-nerve tissues. The effect occurs within two hours and lasts for six to eight hours, with no observable effect on nerve activity or animal behavior.
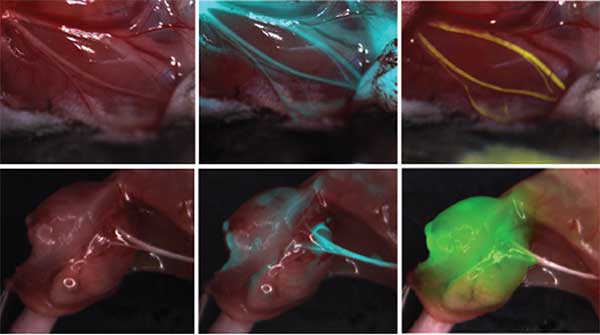
In a mouse model, injectable fluorescent peptides make hard-to-see peripheral nerves glow, alerting surgeons to their location and extent. Left: mouse nerves without fluorescent labeling. Middle and right: labeled mouse nerves.
This fluorescence labeling works even in nerves that have been damaged or severed, as long as they retain a blood supply. In other words, fluorescence labeling might be a useful tool in future surgeries to repair injured nerves.
“The analogy I use is that when construction workers are excavating, they need a map showing where the existing underground cables are actually buried, not just old plans of questionable accuracy,” Tsien said in 2011. “Likewise, when surgeons are taking out tumors, they need a live map showing where the nerves are actually located, not just a static diagram of where they usually lie in the average patient.”
So far, these fluorescent probes have only been tested in rodents. But Nguyen and team are rapidly translating their discoveries into the clinic. First, they found and optimized a novel peptide version that specifically labels nerves in human tissue samples. Then, she and colleagues started a company, Alume Biosciences, to license the nerve-lighting technology from UC San Diego. They are currently conducting the pharmacology and toxicology tests required by the U.S. Food and Drug Administration before they can initiate a clinical trial. Their first test will be in patients with salivary gland tumors undergoing surgery where the facial nerve is at risk for inadvertent injury.
“If all goes smoothly,” Nguyen said, “we could begin testing these nerve-labeling fluorescent probes on human facial nerves in late-2018.”
Revealing memories
While Nguyen’s team uses Tsien’s fluorescent technology to see better with the naked eye, his probes also make it much easier to see cells and their components under microscopes.
“Microscopy evolved as labeling methods evolved,” said Mark Ellisman, professor of neurosciences and bioengineering.
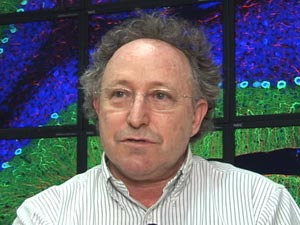
Mark Ellisman, professor and director of the National Center for Microscopy and Imaging Research (NCMIR) at UC San Diego School of Medicine
Ellisman joined the UC San Diego School of Medicine faculty in 1977. In 1988, he founded what is now the National Center for Microscopy and Imaging Research (NCMIR). Supported by the National Institutes of Health, NCMIR is now the go-to place for researchers around the world who want to develop and apply microscopy techniques to look at cellular life at just about any scale.
At NCMIR, you can find more microscopic views than anywhere else in the world. That’s in part because Ellisman and NCMIR scientists are microscopy pioneers. Their facility houses approximately $100 million worth of technology, including electron microscopes, analytical microscopes, even robots that can slice sections of tissue and scan it continuously over weeks to construct 3D images.
These microscopes don’t exactly just come straight from a factory floor. NCMIR staff are constantly building and tinkering to improve them, often contracting with manufacturers. As a result, many of their microscopes are one-of-a-kind.
“The first thing we do when we get a new scope is pop open the hood and fix it up,” Ellisman said. “In many ways NCMIR is like a specialty garage that soups up muscle cars for maximum performance.”
One room-sized microscope, for example, has a hole cut in the back and an additional lens added — a lens made by the same company that made the corrective optics to fix the Hubble telescope. The scope can provide resolution down to one angstrom, the size of one hydrogen atom.
“But we’re not just interested in the technology, we also want to understand how the biology works,” Ellisman said.
To this end, NCMIR takes on an average of 100 projects per year, working with researchers interested in everything from HIV to cancer. With NCMIR technology, researchers can watch how tumor cells migrate, right down to the whiskers on an individual cell’s molecular feelers or “arms” as it reaches and spreads, and how that cell responds to drugs.
According to Ellisman, they pick projects where there is a gap in knowledge. Then the team determines how to further advance probe technology, enhance microscopes, or apply a series of microscopies, to answer the question.
For years, one question has tenaciously nagged at biologists and researchers like Tsien: How are lifelong memories stored?
Shortly before he died, Tsien and his team were working with Ellisman and NCMIR colleagues to find an answer. They wanted to visualize and understand previously underappreciated structures in the brain called perineuronal nets. These nets encase synapses, the structures through which neurons communicate. Tsien believed perineuronal nets play a role in how we store lifelong memories.
One clue is that while many proteins in the body turn over regularly, recent findings by the group show that perineuronal net components are surprisingly long-lasting. Stable isotope labeling experiments and visualization with NCMIR’s advanced electron microscopes and ion microscopes elsewhere are revealing new details about these nets. They may stick around for a mouse’s entire life, as Tsien postulated. And during that time, synapses may form through the net holes, establishing stability and retaining long-term information.
Remaining members of Tsien’s team and NCMIR researchers are now continuing their quest to track how fluorescently labeled perineuronal nets change over time and appear under a variety of conditions, including those that occur in the aging brain.
“This was Roger’s vision and we’re dedicated to carrying it on,” Ellisman said.
This is the third in a four-part series celebrating the 50th anniversary of the UC San Diego School of Medicine. See part 1 and part 2.
Share This:
You May Also Like
Stay in the Know
Keep up with all the latest from UC San Diego. Subscribe to the newsletter today.