Pharming the Microbiome
The collective communities of microorganisms within each of us alter the way drugs work, for good and ill, and may be a source of new medicines themselves if we can parse their multitudinous mysteries.
Published Date
Article Content
At the turn of the 20th century, German physician-scientist Paul Ehrlich was busily transforming medicine: finding a cure for syphilis and a way to stain bacteria for identification purposes, providing a name for the concept of chemotherapy and popularizing the notion of a therapeutic “magic bullet.”
Ehrlich’s contributions still resonate, perhaps most notably the ideas that it is possible to design and dispense pharmacological compounds that will target and kill specific types of unwanted cells, from pathogens to cancer, without harming healthy cells. A magic bullet, so to speak.
But new scientific disciplines, such as genomics, proteomics, metabolomics and microbiomics, have revealed that life is far more complicated than Ehrlich and others might have imagined, and so too, the conditions that ail us. Modern medicine needs a lot more bullets.
It is a myth that bacteria and other microbes in our bodies outnumber our own cells 10 to 1. Nonetheless, the human body is extraordinarily bounteous in microbial life, consisting of approximately 30 trillion “human” cells and another 40 trillion or so nonhuman microbial cells.
The typical person is more microbe than human, and in their entirety, these microorganisms account for an estimated 2 to 6 pounds of average human body weight. Genetically, the numbers are even more dramatic. The human genome consists of roughly 20,000 genes; the human microbiome expresses 2 to 20 million genes. Even within what is usually considered the “human” genome, 8% of human DNA consists of viral remnants; another 40 percent may have viral origins.
“We hear a lot about how our own human genes influence our health and behaviors, so it may come as a shock to think that we could have molecules in the body that look and act the way they do not because of our genes, but because of another living organism.”
Our microorganisms conduct the business of our lives. They digest food; maintain pH levels in saliva, bile, gastric acid and tears; remove dead cells so that live ones can take their place; and colonize every available nook and cranny, inside and out. Our mouths harbor more than 700 species of bacteria and our skin perhaps 1,000 species, plus assorted viruses, fungi, yeast and other forms of life.
Resident microbes determine biological function. In 2020, Pieter Dorrestein, PhD, professor and director of the Collaborative Mass Spectrometry Innovation Center in the Skaggs School of Pharmacy and Pharmaceutical Sciences at UC San Diego, and colleagues published a Nature paper reporting that as much as 70% of a mouse’s gut chemistry is determined by its gut microbiome.
Even in distant organs, such as the uterus or the brain, it was estimated that approximately 20% of resident molecules are influenced by gut microbes.
“We hear a lot about how our own human genes influence our health and behaviors, so it may come as a shock to think that we could have molecules in the body that look and act the way they do not because of our genes, but because of another living organism,” Dorrestein said.
From the moment of birth — indeed from the manner of birth — our microbiomes change with exposure to the world and people. Microbiota are altered by what we eat, where we live and even how we sleep. By age 3, each human being is a unique collection of microbial communities — distinct sites on our bodies as different in terms of their microscopic residents as San Diego soil is from San Diego River water.
“While we are only approximately 0.1% genetically different within our human genome, we can be up to 90% different in terms of our microbial genomes,” observed Rob Knight, PhD, professor and director of the Center for Microbiome Innovation in the Jacobs School of Engineering, with colleagues, in the 2019 edition of Concepts and Principles of Pharmacology.
For the most part and most of the time, human microbiota live in harmony with their hosts. Nearly everyone carries pathogens — microorganisms known to cause illness — but in healthy individuals, these pathogens tend to coexist harmlessly.
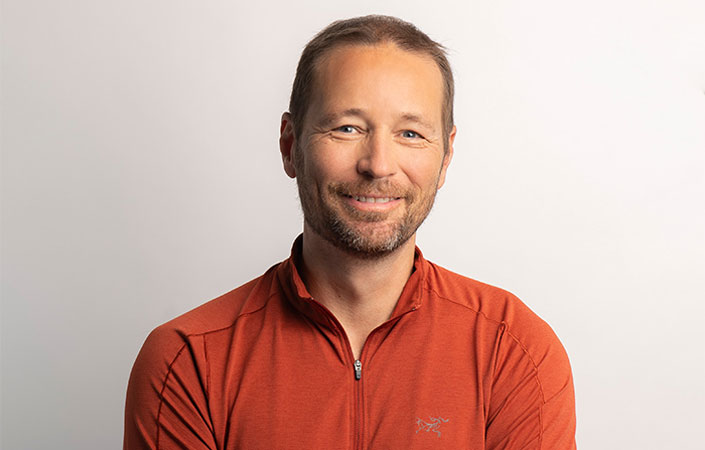
It is a dazzlingly complicated interspecies relationship. Apart from the sheer number of players involved is the even greater number of interactions between them, which includes the trillions of metabolites (molecules generated by the process of metabolism) created by biochemical reactions within cells. By some estimates, this endless production works out to 37,000 billion billion (37 followed by 21 zeros) chemical reactions per second in the human body.
In recent years, much effort has been expended to understand better how the almost unlimited elements of the microbiome and their work products cause disease. Why, for example, does a previously unthreatening pathogen turn deadly? How do individual microbiomes keep people healthy, even as some necessary medications or treatments do quite the opposite? Can we find new magic bullets in the microbiome?
“While we are only approximately 0.1% genetically different within our human genome, we can be up to 90% different in terms of our microbial genomes.”
Answers are coming. Doctors now know that the microbiome plays an important role in whether a patient will respond to some forms of chemotherapy for cancer or suffer adverse effects. Irinotecan is a type of chemotherapy for treating colon cancer, but when metabolized by some species of gastrointestinal bacteria, it causes severe diarrhea. A better understanding of an individual’s microbiome — and more precisely what is residing in the gut — would help doctors stratify which patients are most likely to benefit from a drug such as irinotecan and which will likely be worse off.
There are increasing efforts to leverage the microbiome to more directly restore or promote health. The most notable case, perhaps, is fecal microbiota transplantation (FMT) to treat infections of Clostriodioides difficile, a bacterium that causes severe diarrhea and inflammation of the colon and is resistant to most antibiotics.
FMT is celebrated as one of the first and most high profile examples of successful bacteriotherapy. It involves the transfer of stool from a healthy donor into the gastrointestinal tract of a patient with recurrent C. difficile colitis. The intent is to replenish and restore a healthy bacterial balance. “It’s a key example to date of a clinical application for commensal microbes as live biotherapeutic agents,” wrote Knight and colleagues.
FMT is now considered a standard of care for C. difficile colitis. It has an estimated cure rate of 85% to 95%, according to published data, though treatment may require multiple applications.
Other bacteriotherapy efforts are more literally more superficial, but equally encouraging. Richard Gallo, MD, PhD, Distinguished Professor and founding chair of the Department of Dermatology at UC San Diego School of Medicine, and colleagues are developing a topical treatment derived from skin bacteria for atopic dermatitis, the most common form of eczema.
Atopic dermatitis causes red and itchy skin, and afflicts nearly 10 million children and more than 17 million adults in the U.S.
In February 2022, Gallo and colleagues published a study describing a universal strain of bacteria derived from healthy human skin that, when applied in a lotion to the skin of patients with atopic dermatitis, resulted in the introduced harmless bacteria inhibiting the growth of Staphylococcus aureus, a pathogenic bacterium that aggravates skin conditions, including eczema. Clinical trials are ongoing.
The human gastrointestinal tract is the most-studied of microbiomes.
It is rich in diversity and abundance — an estimated 1 billion bacteria per milliliter of content representing up to 1,000 possible species in the colon alone — and strongly linked to both health and disease.
One of the first discoveries linking microbiota to a human ailment involved inflammatory bowel disease (IBD). The causes of IBD aren’t fully understood, but it’s clear that the chronic condition is moderated by gut microbiota: too much or too little. Too many of the “wrong” bacterial species and not enough of the “right” all play a part, influenced by factors such as genetics, diet, drugs and stress.
Antibiotics are celebrated as a marvel of modern medicine. They represent one of the most successful forms of chemotherapy in history, effectively treating a vast array of diseases in the 20th century and dramatically extending the human lifespan.
But apart from their diminishing returns due to evolving antimicrobial resistance, broad-spectrum antibiotics pose peril to the human microbiome, killing not just targeted pathogens, but also harmless and beneficial microbes. Not only does prolonged or indiscriminate use of antibiotics reduce overall microbial diversity (not a good thing), it opens the door and space for opportunistic pathogens such as C. difficile and may lead to greater risk of autoimmune and metabolic diseases, such as diabetes, asthma and allergies.
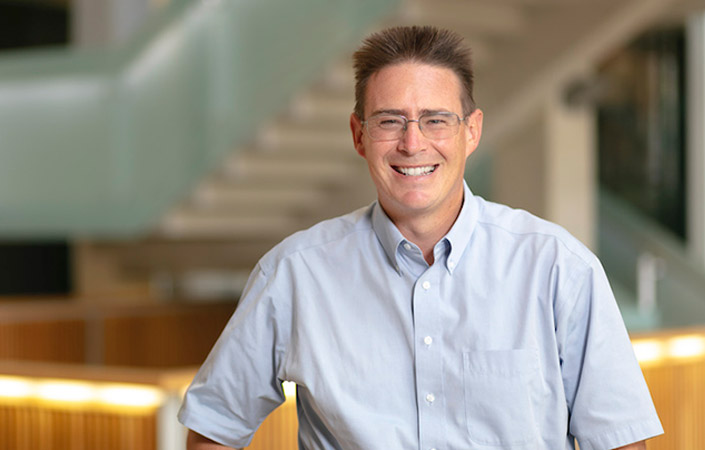
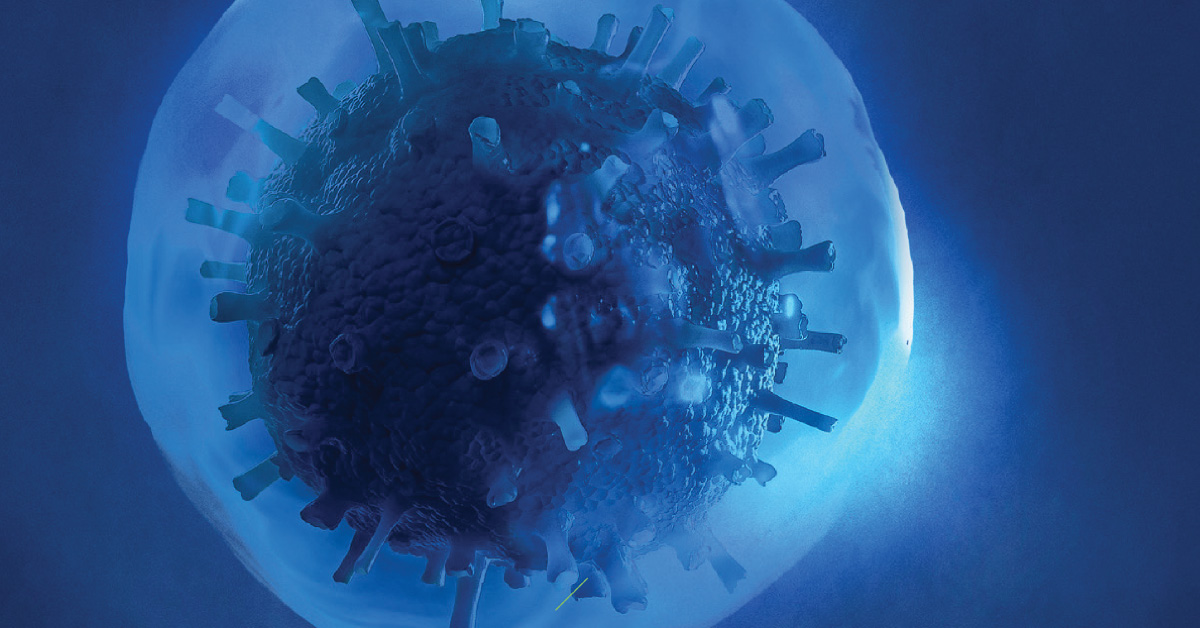
The gut microbiome plays a role, too, in cardiovascular disease by altering how nutrients are broken down. In some cases, it appears, these changes lead to dysregulated metabolic aspects of cholesterol and elevated heart disease risk.
And then there’s the “gut-brain axis,” a two-way signaling network between the gastrointestinal tract and the central nervous system in which gut microbiota are major players. Studies have found that alterations in gut microbiota are associated with anxiety and depression because their signaling molecules act as sensory information transmitted to the brain. Neuroimaging suggests that an abundance of certain bacterial taxa in the gut, and its resulting byproducts, may influence neurological disease.
A 2020 study by Olivier George, PhD, professor of Psychiatry at UC San Diego School of Medicine, and colleagues reported that antibiotic treatment and depleted gut microbiota in rats dramatically changed how their brains functioned during opioid addiction and withdrawal.
“The most surprising thing was that the rats all seemed the same on the surface,” said George when the study was published in eNeuro. “There weren’t any major changes in the pain-relieving effect of opioids, or symptoms of withdrawal or other behavior between the rats with and without gut microbes, but in the end, it became clear that, at least in rats, gut microbes alter the way the brain responds to drugs.”
Less is known about other non-antibiotic drug classes, though there are increasing reports that other medications also alter the composition of the gut microbiome to good and bad effect.
Metformin, for example, is widely prescribed to treat diabetes, but its impact on the gut microbiome is felt too. Proton pump inhibitors, commonly used to reduce stomach acid and heartburn, also reduce and change gut microbiome diversity, elevating the risk of C. difficile infection.
Conversely, gliptins, a class of antidiabetic agents used to control blood sugar levels, have been found in mouse studies to inhibit some pathogenic types of gut bacteria, improving microbiome health.
The efficacy of any drug largely depends upon how well it is metabolized by the body or by targeted parts of the body. A medication that works for one person doesn’t necessarily work for another with the “same” condition.
Metabolism is highly variable among individuals, influenced to some degree by genetic variation, which again largely means the particular mix of microorganisms living within them.
For example, microbes help determine how drugs degrade in the human body. Some contribute to the activation of medications, such as sulfasalazine, a rheumatoid arthritis drug that requires a bacterial enzyme to help turn it on.
Other microorganisms inactivate drugs, reducing their bioavailability and usefulness. Patients with cardiac arrhythmia and heart failure may be prescribed digoxin, a therapeutic that must be maintained at specific levels in the bloodstream to be effective. However, certain bacterial strains metabolize digoxin, rendering it inactive and the patients at greater risk if levels drop.
The microbiome can also limit the therapeutic benefit of drugs by simply using the same metabolic pathways. Some patients are at higher risk of liver damage caused by acetaminophen, the active ingredient in many pain relievers, because their gut microbiota have reduced the ability to metabolize it elsewhere.
Our microbial partners offer a source of new drugs. Nearly half of all FDA-approved human pharmaceuticals originate from natural sources. Aspirin famously evolved from a compound found in the bark and leaves of the willow tree; penicillin, lovastatin and Taxol also derive from terrestrial plants and microbes.
But more than two-thirds of the planet is covered by ocean, said Bradley Moore, PhD, Distinguished Professor at Skaggs School of Pharmacy and Pharmaceutical Sciences and Scripps Institution of Oceanography, making it a vast and rich repository of marine-derived pharmacopoeia, especially microbial.
Marine microorganisms comprise an estimated 70% to 90% of the oceans’ biomass. A single liter of seawater may contain 1 billion bacteria and 10 billion viruses
Moore and colleagues at the Center for Marine Biotechnology and Biomedicine at Scripps Institution of Oceanography are investigating scores of aquatic sources, from algae to sea slugs to deep-sea microbes, for new medications.
Two cancer drug candidates currently in Phase III clinical trials — marizomib and plinabulin — are derived from marine bacteria and fungi discovered and developed by William Fenical, PhD, and Paul Jensen, PhD, both at Scripps.
“These examples showcase the remarkable promise of marine microbes and their distinctive chemical language in providing new opportunities to treat disease,” said Moore, who with colleagues has reported promising marine-derived pharmaceuticals from organisms ranging from cyanobacteria and sponges to soft corals, all of which may have therapeutic applications as antibiotics, antivirals or anticancer agents.
Other scientists are looking within for remedies. New abilities to mine the human microbiome are allowing researchers to identify natural microbial products analogous to known drugs. Like microbes everywhere, human microbiota must compete for finite space and resources and so have evolved a variety of tools to survive, including quorum quenching molecules that disrupt signaling and growth of other microbes. These molecules could be exploited to treat biofilm infections—colonies of mixed or single bacterial types that are particularly resistant to antibiotics and other threats.
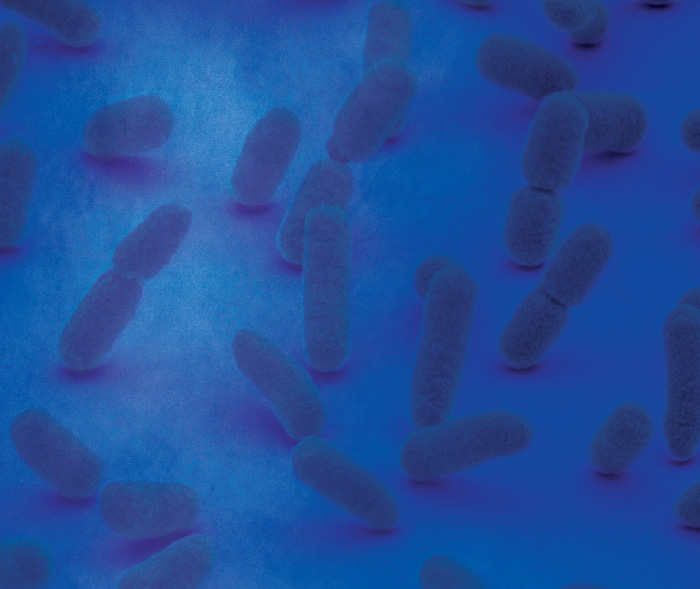
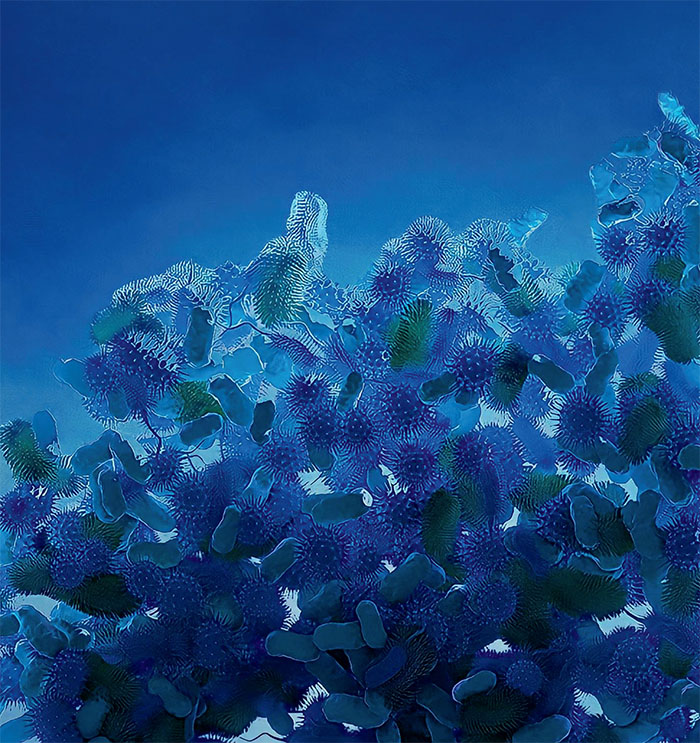
Beneficial bacteria known as probiotics and the foods they eat (prebiotics) could be tapped as well, and there are a number of efforts underway to use them to treat conditions from oral and allergic diseases to major depressive disorder.
In a study published in the Journal of Integrative and Complementary Medicine, for example, Christine Tara Peterson, PhD, assistant project scientist in the Department of Family Medicine at UC San Diego School of Medicine‘s Center of Excellence for Research and Training in Integrative Health, and colleagues described the prebiotic potential of herbal medicines.
They found that three herbal medicines commonly used in Ayurveda for the treatment of gastrointestinal health and disease alter the growth and abundance of specific bacterial species, which may improve colon function, reduce inflammation and protect against opportunistic pathogens.
“Medicinal herbs are chemically complex, much more than the word ‘herb’ may commonly conjure,” said Peterson. “Ayurvedic herbs potentially yield a host of medicinal properties with far-reaching effects on multiple targets and body systems.
“I am working within the scope of a new emerging model whereby herbal medicine and product development move beyond a single target or traditional pharmaceutical molecule approach to an expanded view that includes the microbiota-mediated therapeutic effects of herbal medicines and allows for the potential of multiple molecules of interest (both herb-derived and microbe-generated) to better explore complex, multi-target and multisystem responses to traditional herbal medicine.”
Finally, it may be possible to engineer existing commensal microorganisms to perform better or differently. Some strains of Escherichia coli bacteria are notorious for causing intestinal distress, urinary tract infections and, sometimes, life-threatening kidney failure, but most E. coli species reside naturally and harmlessly in the gut where they, at very least, prevent colonization of the intestines by more dangerous microbes.
Researchers have engineered commensal E. coli to sense and disperse biofilms created by Pseudomonas aeruginosa, a pathogenic bacterium that causes serious disease, including pneumonia and septic shock.
E. coli bacteria have also been genetically modified to produce phenylalanine-metabolizing molecules in animal models of phenylketonuria or PKU, a rare inherited disorder in which accumulation of the amino acid phenylalanine can lead, without treatment, to severe brain damage.
Last year, the National Institutes of Health launched a new consortium called Nutrition for Precision Health. Part of that effort includes a Microbiome and Metagenomics Center at UC San Diego to analyze the microbiomes found in stool samples of study participants, based on the idea that what we eat affects our gut microbiomes which, in turn, affects our overall health.
“Bringing this expertise and technology to bear on the incredibly challenging problem of nutrition and health will enable a whole new level of precision in answering the age-old question of ‘what should I eat today’?’” said Knight. “We are just starting to understand how the microbiome can answer this with a surprising level of individual detail, not just broad-strokes generalizations for the whole population.”
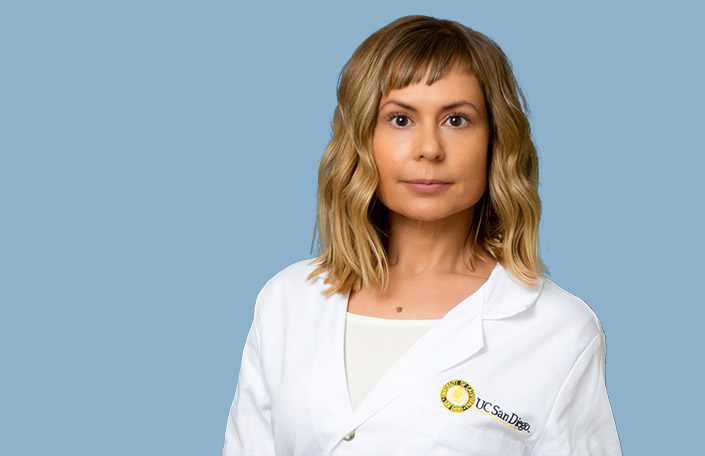
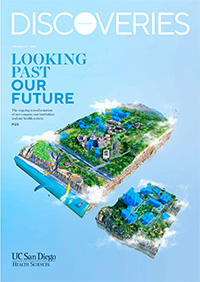
About Discoveries Magazine
This article was originally featured in the 2023 issue of Discoveries, the annual magazine from UC San Diego Health Sciences. Discoveries highlights the latest and greatest in the university's health science research, education and clinical practice. Other stories in this issue explore topics such as mindfulness meditation, wearable medical devices and a profile of new Vice Chancellor for Health Sciences, John M. Carethers.
Share This:
You May Also Like
Stay in the Know
Keep up with all the latest from UC San Diego. Subscribe to the newsletter today.