New Technology from UC San Diego Cracks Fundamental Challenge in Neuroscience
Researchers monitored single-neuron activity from hundreds of neurons along with the local network activity in the brains of mice
Published Date
Story by:
Media contact:
Share This:
Article Content
A new study by researchers at the University of California San Diego demonstrates a new neurotechnology geared toward answering a long-running question in neuroscience: How can scientists link the separate activity of many single neurons in the brain to the waves and oscillations of the local broader network?
The study introduces E-Cannula, a new technology that enables simultaneous electrical recording and optical imaging of hundreds of neurons on a 2D plane in the brains of mice. It represents a long-awaited neuroscience breakthrough that enables the study of population-level neuronal dynamics such as brain waves, rhythms, and oscillations, along with single neuron activity for a large population of neurons from the same brain region.
Using this technology, the researchers show that certain high-frequency rhythms, or sharp wave ripples, can selectively recruit different cell assemblies in the hippocampus, an area of the brain that is crucial for learning and navigation.
The researchers published their findings on Oct. 4 in Cell Reports. The work is from the lab of UC San Diego electrical engineering professor Duygu Kuzum, in collaboration with the Attila Losonczy Lab from Columbia University.
“E-Cannula is a versatile technology that could be used to uncover unknown aspects of the population-level neural dynamics across brain regions to study brain computation at the system level,” said corresponding author Duygu Kuzum, a professor of electrical and computer engineering at the UC San Diego Jacobs School of Engineering. “It also helps us investigate neural network dysfunctions that can lead to various brain disorders.”
-Cannula integrates flexible transparent microelectrodes with an imaging cannula implanted in the brain. The transparent and flexible microelectrodes use monolayers of graphene, which are transparent, low-noise and extremely flexible. The graphene electrodes and wires used to monitor neural signals are patterned using microfabrication techniques. Two layers of transparent and flexible polymer encapsulate the graphene wires to electrically insulate the device and provide protection for long-term in vivo experiments. The flexibility of the transparent microelectrodes allows the wires to bend up to 90 degrees and conform with the steep sidewalls of the imaging cannula. Transparent microelectrodes show stable electrical recording capability after mechanical stress is applied through 120 bending cycles.
“Understating population-level dynamics in the brain and linking them to cellular spikes requires a new technology that enables both recordings. E-Cannula does that by allowing simultaneous electrical recordings and imaging of neurons in the same exact neuron population in the brain,” said Mehrdad Ramezani, co-first author of the study, a graduate student in the Department of Electrical and Computer Engineering at the UC San Diego Jacobs School of Engineering.
E-Cannula can be used for imaging and electrical recording of hundreds or thousands of neurons from the same location of the brain. This has not been feasible with any other existing technology. Other approaches implant electrical recording probes in one hemisphere of the brain while imaging the neural activity from the other side using multiphoton microscopy. E-Cannula combines imaging and recording on the same hemisphere to concurrently image and record from the same population of neurons.
Exploring fundamental neuroscience questions
The project began with a desire to understand how different brain rhythms or oscillations can selectively recruit different neurons to perform computations related to learning and navigation. Certain sets of neurons in the hippocampus, known as place cells, encode spatial information during navigation. They fire when an animal visits specific regions of its environment. Place cells are thought to provide the foundation for an internal representation of space, also known as a cognitive map.
How the neurons are recruited during learning or navigation and the roles of brain rhythms or oscillations in the selective recruitment of cell assemblies have long been topics of curiosity for neuroscientists. Because it has been difficult to study cellular activity from hundreds of cells with brain oscillations simultaneously recorded locally from the same populations, such questions have been difficult for neuroscientists to study head-on.
“We were interested in investigating these questions, so we built a technology to explore this neuroscience problem,” Kuzum said.
The researchers implanted E-Cannula in the hippocampus of transgenic mice and explored the relationship between sharp-wave ripples and the spiking activity of neurons from the same areas. They found that the specific characteristics of these oscillations are associated with the synchronous activity of pyramidal neurons in the hippocampus.
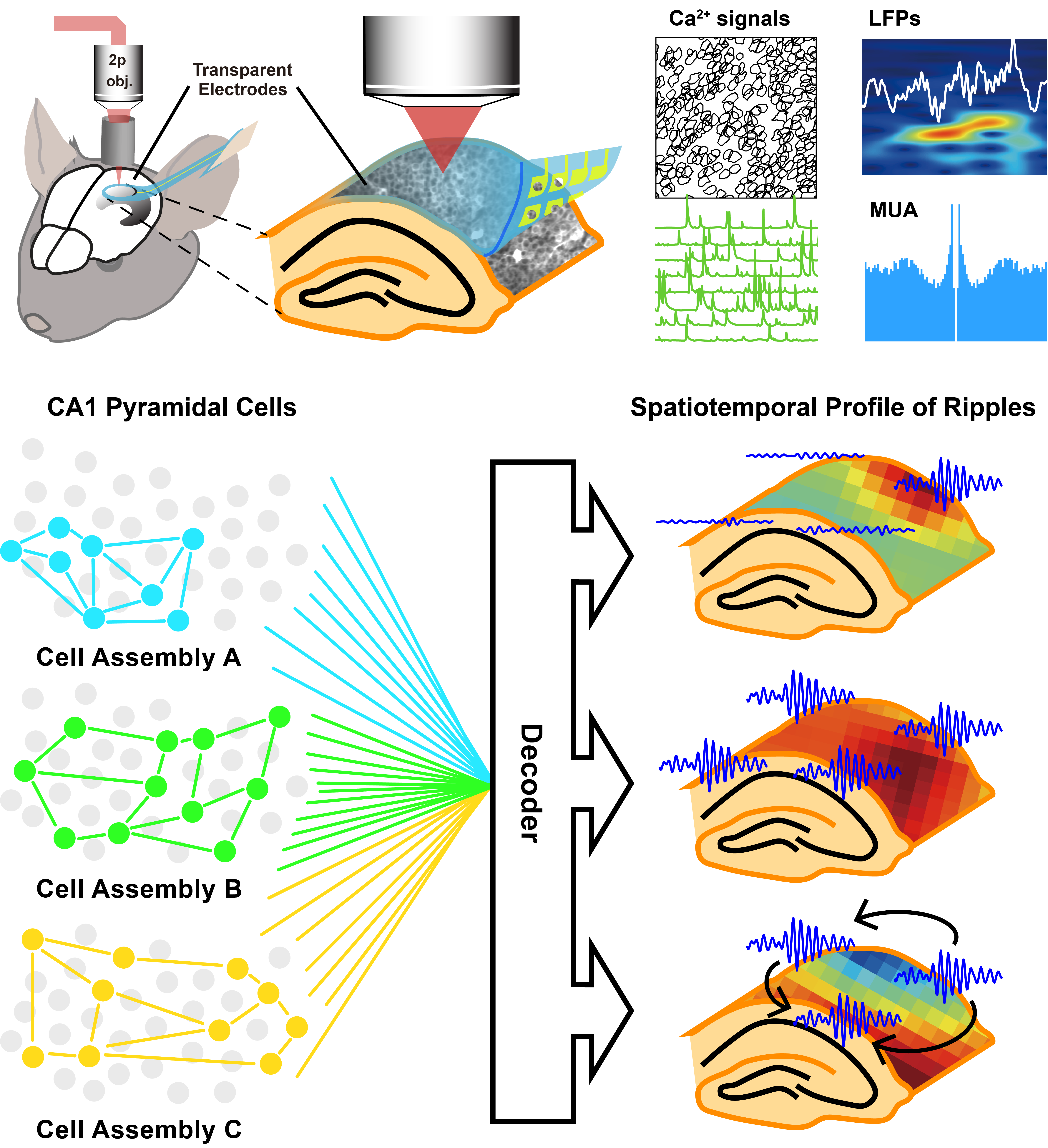
Experiments with E-Cannula revealed that hippocampal ripple waves are associated with the selective recruitment of orthogonal cell assemblies. Results suggest that the diversity in spatio-temporal patterns of ripple waves, such as whether they are classified as stationary or traveling, or local or global, may serve as a mechanism for the selective activation of the unique hippocampal cell assemblies associated with the encoding of distinct memories.
“That can potentially help us to better understand how the biological brain can learn continually, life-long, without going through catastrophic forgetting, a major shortcoming of artificial neural networks used in AI today,” said Kuzum. “We hope to translate these findings to theoretical frameworks that can benefit artificial intelligence research.”
Paper title: "E-Cannula reveals anatomical diversity in sharpwave ripples as a driver for the recruitment of distinct hippocampal assemblies ," in Cell Reports. Co-authors of the study include Xin Liu, Jeong-Hoon Kim, Yichen Lu at UC San Diego and Satoshi Terada, Andres Grosmark, Attila Losonczy at Columbia University.
This research was supported by grants from the Office of Naval Research (grant nos. N00014161253 and N000142012405);, the National Science Foundation (grant nos. ECCS-2024776 and ECCS-1752241); the National Institutes of Health (grant nos. R21 EY029466, R21 EB026180, and DP2 EB030992); the JSPS Overseas Fellowship; National Institute of Mental Health grants 1R01MH124047 and 1R01MH124867; National Institute of Neurological Disorders and Stroke grants 1U19NS104590, 1U01NS115530, and 1R01NS121106; and the Kavli Foundation. Fabrication of the electrodes was performed at the San Diego Nanotechnology Infrastructure of UC San Diego, a member of the National Nanotechnology Coordinated Infrastructure, which is supported by the National Science Foundation (NSF; grant no. ECCS-1542148).
Share This:
Stay in the Know
Keep up with all the latest from UC San Diego. Subscribe to the newsletter today.