Discovering the Magic Number of Earth Ice Molecules
UC San Diego researchers lead international team in chemistry milestone
Published Date
By:
- Lisa Petrillo
Share This:
Article Content
It took over two years, 10 scientists, multiple laser blasts, the muscle of a supercomputer and innovative lab bench work. And so armed, an international team, including researchers from the University of California San Diego, the University of Utah and the University of Göttingen, has cracked the mystery of a decades-old chemistry problem: How many molecules does it take to make ice on Earth?
And their answer is … 90 molecules.
The results of this research appeared recently in the Proceedings of the National Academy of Sciences of the United States of America (PNAS), the journal of the National Academy of Sciences, in a paper entitled, “The end of ice I.”
As old as Earth is, and as much studied as water has been as the elemental planetary building block, chemists have long been debating the smallest number of water molecules capable of freezing into ice. Previous studies estimated that between 200 to 1,000 molecules are necessary to form the smallest ice crystal, explained paper co-author Francesco Paesani, UC San Diego Professor in the Department of Chemistry and Biochemistry and chemistry postdoctoral researcher Daniel Moberg, the paper’s lead author.
“It's like a textbook discovery, that ice cannot form if you have fewer than 90 molecules,” said Paesani, a theoretical chemist who is also affiliated with the Department of Materials Science and Engineering in the Jacobs School of Engineering and the San Diego Supercomputer Center. “We were very happy because we were able to combine theory and experiment and narrow down the size of the smallest ice crystal.”
That would be 90 molecules of ice on Earth, technically known as ice I. There are some 18 known kinds of ice—the crystalline kind—existing mostly in either space or theory. For this 90-molecule-minimum ice I, they’re talking the kind of ice you skate on and chill your lemonade with.
The researchers discovered their 90-molecule answer both computationally and experimentally by breaking down the process to the quantum level. As Moberg notes, an ice crystal of 90 molecules is too small to see, at less than 3 nanometers wide, even with microscopic aid. Even smaller than a snowflake.
“Other papers have gone down that small before, but they were not paired with experiments,” said Moberg. “We were happy to find this number at that small of a size and to back it up both with theory and experimental evidence.”
In addition to solving the mystery number of molecules that turns water into ice, the researchers further distinguished their work by their methods. They combined both traditional bench scientific methods with theory and new laser and infrared spectroscopy (IR) techniques, allowing deeper analysis of how light and matter interact. While both IR and chemistry theory tools used for the research are not new, the international team theorized novel ways to generate and measure the water clusters that made their experiments possible in the lab.
As noted in the paper, only in the last decade have IR techniques advanced to the point where the appearance of characteristic ice I signatures can be observed with precise size resolution, first in neutral clusters and later in doped (treated) clusters. What took so long to crack the ice freezing threshold mystery was the complexity of working in the quantum world, Paesani explained, who believes the results validate the powerful level that computer modeling has achieved.
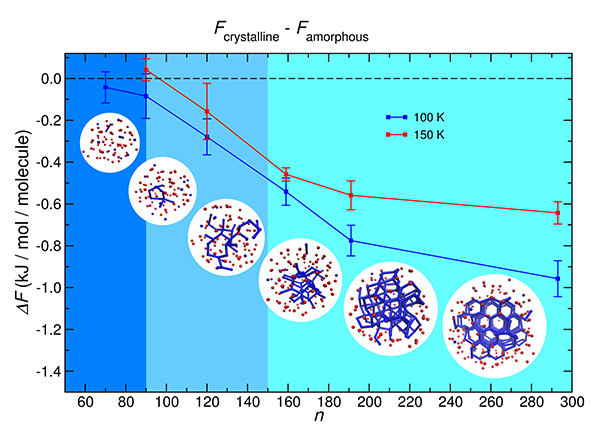
An international team led by UC San Diego theoretical chemists successfully captured the lowest threshold of freezing water into ice, at 90 molecules, and tracked the growing numbers of molecules crystalized to freezing levels in a snowball effect. Graphic and video (at top) by “The end of ice I” research team
“This implies that we can also use computers in the same way to model reality with a level of realism that is quite remarkable—we can use computers to actually describe what is happening in nature. That's why I find this part of the work very fascinating,” he said.
Paesani’s research group at UC San Diego addresses problems at the intersection between chemistry, physics and computer science. He trains postdoctoral researchers, like Moberg, as well as graduate and undergraduate students to integrate their chemistry knowledge with the fundamental laws of physics that allow chemical transformations to happen, and then translate the resulting theoretical models into efficient computer codes that, he likes to say, help bridge the gap with reality.
“Water is fundamental for life, as we know it. And so being able to reproduce water at the molecular level has always been a fundamental challenge for the entire theoretical and computational chemistry community,” said Paesani, who holds a Ph.D. in theoretical physical chemistry from the University of Rome and has won multiple awards from the National Science Foundation and the American Chemical Society.
Moberg, a former Paesani Research Group member who now works as a postdoctoral researcher at Argonne National Laboratory, experiences ice on a personal level as the lab is located in the Midwest. He said that he finds the oscillating region between ice crystallization and the almost-frozen state the most intriguing aspect of their research.
“We also found a region where what we think of as ice or liquid isn't set in stone, it's oscillating between the two, and you can't pinpoint one or the other,” said Moberg, adding that this could lead to many interesting developments in both climate science or atmospheric science, Interstellar science, biological studies and engineering. “We don't know yet. We're not sure what possibilities or applications this could lead to. But we think it's very interesting and potentially very game changing.”
At those small scales, according to University of Utah chemistry professor and study co-author Valeria Molinero, the transition between ice and water gets a little frizzy.
“The results go beyond just ice and water,” Molinero says, adding that the small-scale phenomena should happen for any substance at the same scales. “In that sense, our work goes beyond water and looks more generally to the coda of a phase transition, how it transforms from sharp to oscillatory and then the phases themselves disappear and the system behaves as a large molecule.”
Other co-authors on “The end of ice I,” are: Daniel Becker, Christoph W. Dierking, Florian Zurheide and Thomas Zeuch of the Institute of Physical Chemistry, Georg-August University of Göttingen; Bernhard Bandow of the Max Planck Institute for Solar System Research, Germany; Udo Buck of the Max Planck Institute for Dynamics and Self-Organization, Germany; and Arpa Hudait of the University of Utah.
This research was supported by the National Science Foundation (NSF), Center for Aerosol Impacts on Chemistry of the Environment (grant no. CHE-1305427), Deutsche Forschungsgemeinschaft (grant ZE890, nos. 1-2, 143238629 and 4-1, 400183980) and the Extreme Science and Engineering Discovery Environment 107, which is supported by the NSF (grant no. ACI-1053575).
Share This:
You May Also Like
Stay in the Know
Keep up with all the latest from UC San Diego. Subscribe to the newsletter today.