By:
- Katherine Connor
Published Date
By:
- Katherine Connor
Share This:
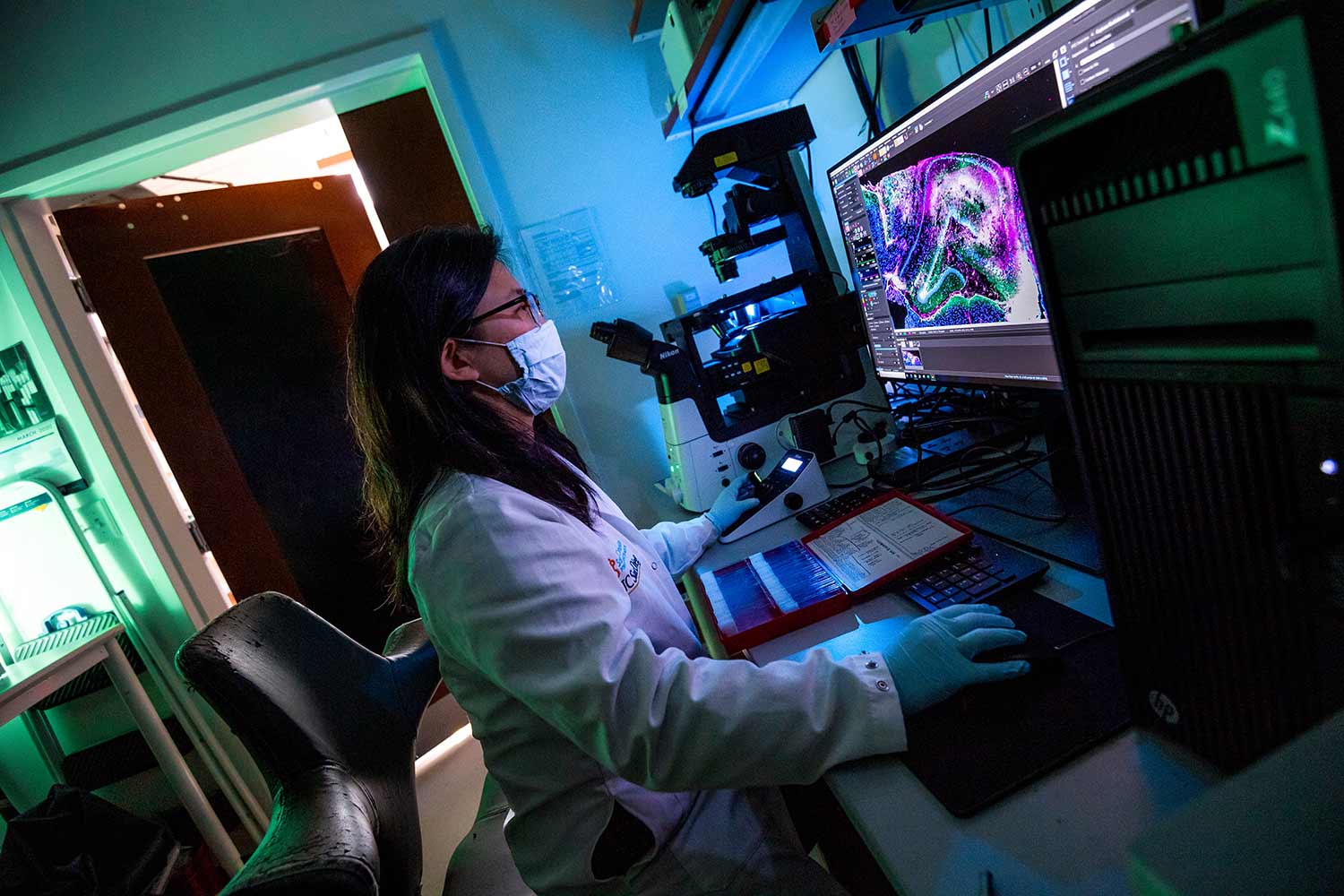
Ester Kwon, a professor of bioengineering at the Jacobs School of Engineering, is developing nanomaterials to diagnose and treat traumatic brain injuries. Photos by Erik Jepsen/UC San Diego
.Breaking Through to the Brain
Engineering nanomaterials to diagnose and treat traumatic brain injuries
Traumatic brain injuries might have faded from the headlines since the NFL reached a $765 million settlement for concussion-related brain injuries, but professional football players aren’t the only ones impacted by these injuries. Each year, between 2 million and 3 million Americans suffer from traumatic brain injuries—from elderly people who fall and hit their head, to adolescents playing sports or falling out of trees, to people in motor vehicle accidents.
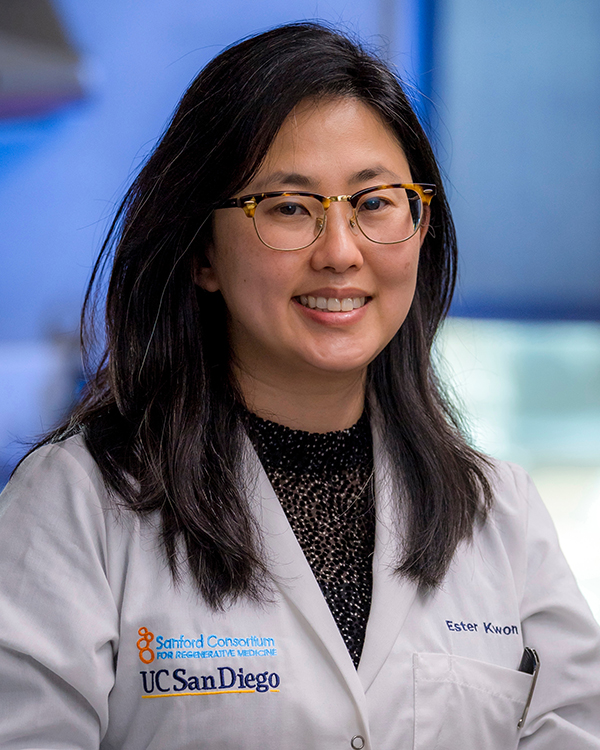
Kwon works at the crux of two burgeoning fields: biological nanomaterials and neuroscience.
There are currently no treatments to stop the long-term effects of a traumatic brain injury (TBI), and accurate diagnosis requires a visit to a medical center for a CT scan or MRI, both of which involve large, expensive equipment.
UC San Diego bioengineering Professor Ester Kwon, who leads the Nanoscale Bioengineering research lab at the Jacobs School of Engineering, aims to change that. Kwon’s team is developing nanomaterials—materials with dimensions on the nanometer scale—that could be used to diagnose traumatic brain injury on the spot, be it a sports field, the scene of a car accident, or a clinical setting. They’re also engineering nanoparticles that could target the portion of the patient’s brain that was injured, delivering specific therapeutics to treat the injury and improve the patient’s long-term quality of life.
“It’s really a dire state for these patients, and TBI is unfortunately quite prevalent,” said Kwon.
“Patients with a traumatic brain injury will often experience changes in their motor function, their cognitive abilities and psychosocial behavior, such as depression.”
Overcoming the blood-brain barrier
Diagnosing and treating brain injuries has stymied scientists and physicians for so long for several reasons, the first of which is the blood-brain barrier. The barrier is a semipermeable layer of cells meant to keep potentially harmful toxins that circulate in the blood from entering the brain. This barrier makes it difficult to get diagnostic or therapeutic particles into the brain.
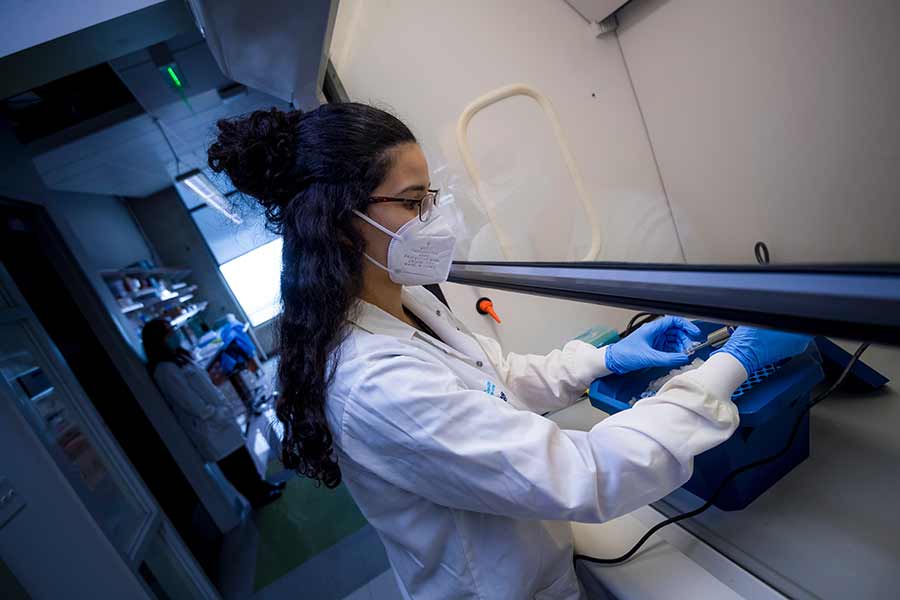
Rebecca Kandell, a bioengineering PhD student in Kwon's lab, is also a Sloan Scholar.
To overcome the challenge of bypassing the blood-brain barrier, Kwon’s lab is taking advantage of a recent insight from the realm of cancer research.
“Nanomedicine has historically been applied to the field of cancer,” said Kwon. “My lab has been thinking about how there are some parallels in disease physiology between brain injuries and cancer. For example, there are changes to the vasculature in a cancer patient—the blood vessels in a tumor aren't normal, they have holes—and people have exploited that to get nanomaterials into the tumor through these holes. Similarly, in TBI, there are holes in the vasculature created by the injury that can be exploited to get nanomaterials into the brain.”
Diagnosing TBI
Kwon’s research group aims to diagnose TBI using biomarkers from a simple urine or blood sample. While scientists have known for decades that a class of enzymes called proteases show increased activity in the injured parts of the brain, getting a sensor into the brain to measure the activity of these proteases remained elusive. Taking advantage of the vascular holes caused by TBI, Kwon’s team was able to develop a nanomaterial that can detect this protease activity, and release a fluorescent signal in the exact location where activity is increased. Their studies, published in February 2020 and December 2021, were the first demonstration of a sensor that could detect protease activity to identify and locate TBI in mice.
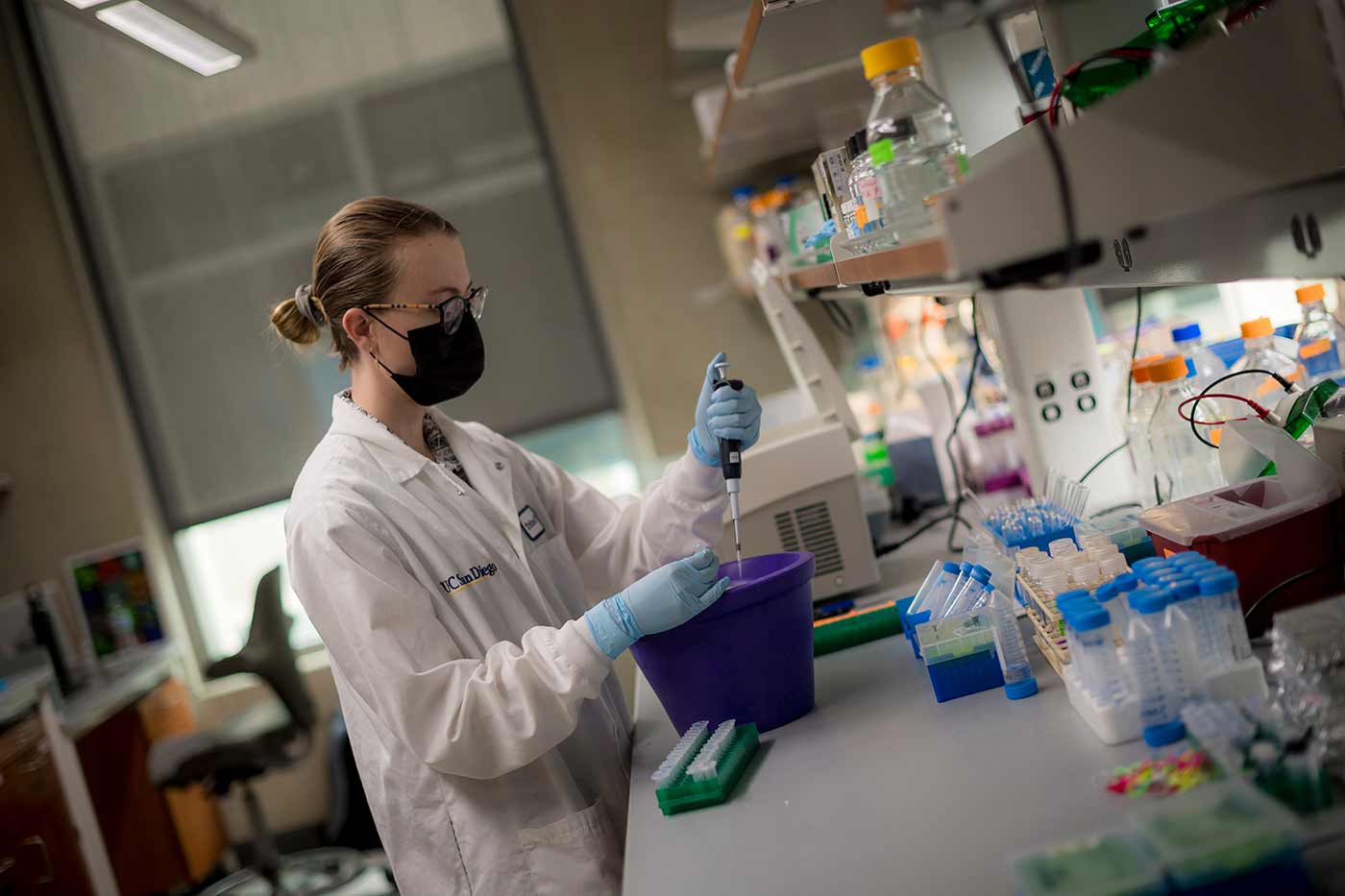
Researchers in Kwon's lab were the first to demonstrate a sensor that could detect protease activity to identify and locate TBI.
Follow-on studies in mice showed that levels of this protease activity picked up by their nanosensors, which were delivered intravenously, could be measured in blood and urine samples.
While researchers still need to perfect the measurement of these protease signals from urine or blood samples before the TBI diagnostic tool could be used by humans, promising advances from a similar concept used for liver fibrosis are encouraging.
“A very similar type of material has already been used in humans to detect liver disease, which is exciting for us in terms of the approach being established as safe,” said Kwon.
Treating TBI
In addition to diagnosing TBI, Kwon’s lab is working to engineer nanoparticles that could be designed to reach the specific part of the brain that was impacted, carrying a therapeutic payload to reduce inflammation, or deliver pro-regenerative therapeutics. These two components would work in tandem, with the diagnostic tool generating information on the exact type and location of the injury, to inform what specific therapeutics would work best and where they need to be delivered.
“Our big dream is to create a precision medicine approach,” said Kwon. “Which is basically to understand the molecular basis for the disease, and apply a therapeutic that’s specific to that molecular underpinning. Because the patient population is heterogeneous, if we know more precisely what’s happening molecularly, we can potentially match therapeutics to their condition and hopefully mitigate any side effects.”
The nanoparticles her lab develops are about the size of a virus. They are made up of a polymer core which carries the desired payload, coated in an outer layer of peptides which give it a location to travel to, like a GPS.
RNA-loaded nanoparticle
In addition to filling the core of these nanoparticles with therapeutic drugs, Kwon’s lab is also working to engineer a nanoparticle that could be used to carry RNA material to the brain for a variety of purposes, from reducing inflammation, to encouraging damaged cell growth. These particular nanoparticles are made from lipids that protect the RNA cargo, and ensure it can be released and activated where needed. This type of lipid nanoparticle carrying RNA cargo is similar to what is used in the mRNA vaccines for COVID-19.
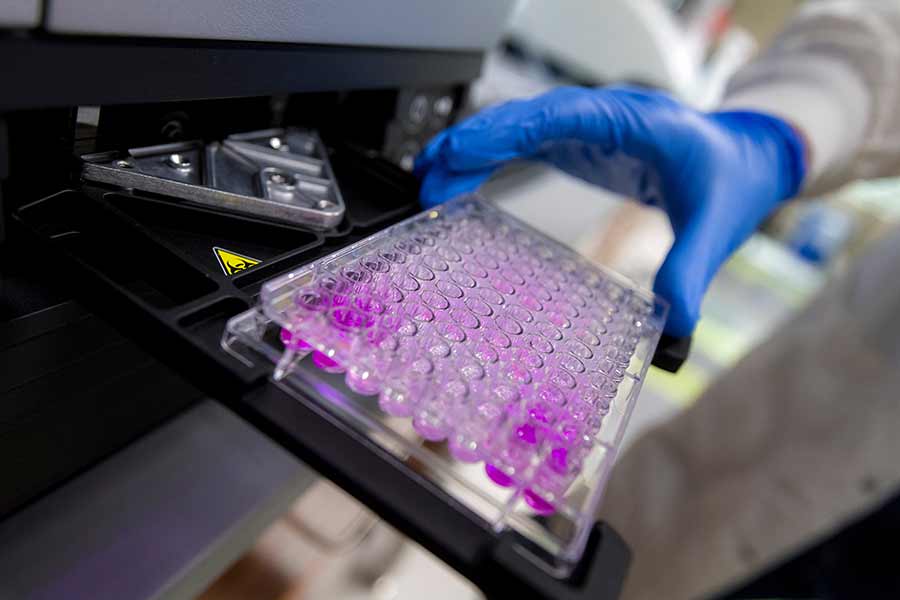
Kwon's lab is also working to engineer a nanoparticle that could be used to carry RNA material to the brain.
To date, there haven’t been any targeted lipid nanoparticles that can reach specific cell types in humans, which would be necessary for use in the brain. Engineering these particles to target specific cell types in the brain is a main focus area of Kwon’s research.
For Kwon, all of the possibilities and experimentation are part of the excitement of working at the crux of the two burgeoning fields of biological nanomaterials and neuroscience.
“In general, the field of nanomaterials for the brain is a few decades behind our sisters and brothers in cancer therapy,” she said. “There has been a lot of rapid development in neuroscience—our knowledge of the basic biology of the brain—which has only happened in the last decade or so. And that sets the table for engineers to start understanding how to design materials to interact with the brain in more sophisticated ways.”
Share This:
You May Also Like
Stay in the Know
Keep up with all the latest from UC San Diego. Subscribe to the newsletter today.